China condemns assassination of Hamas political chief Haniyeh
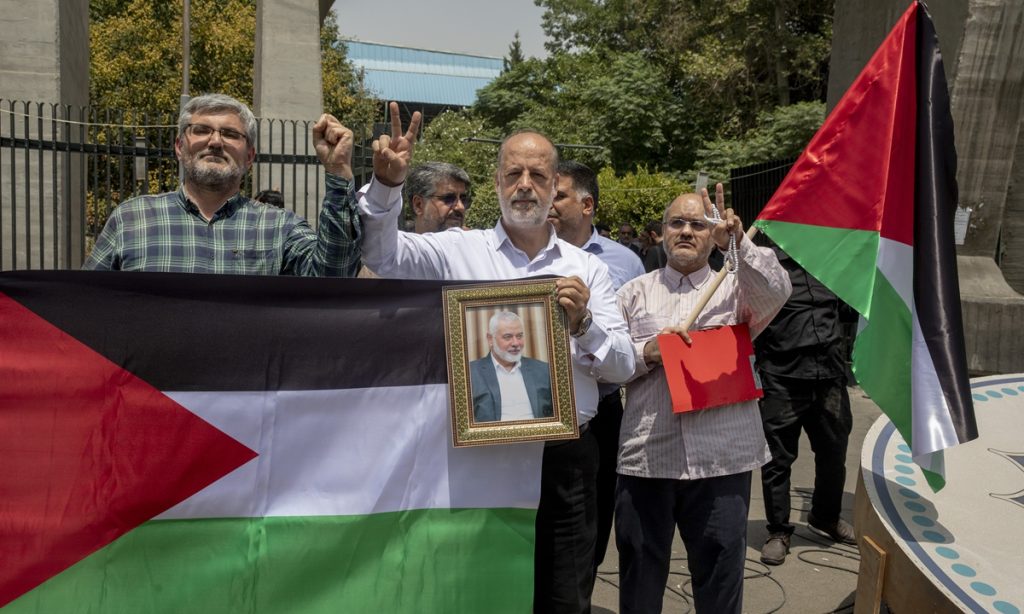
Hamas' political chief, Ismail Haniyeh, was targeted and killed in an Israeli strike early Wednesday while visiting Iran, according to a statement released by Hamas on the same day.
China said on Wednesday that it is closely monitoring the situation, and voiced its firm opposition to and condemnation of acts of assassination.
"We are closely following the incident. China firmly opposes and condemns the act of assassination and is deeply concerned that the incident may plunge the region into greater turmoil. China has been calling for settling regional disputes through negotiation and dialogue and an early, comprehensive and permanent ceasefire in Gaza and there should be no more escalation of the conflict and confrontation," Chinese Foreign Ministry spokesperson Lin Jian said on Wednesday.
Some Chinese experts said that the wave of conflicts in the Middle East is likely to overshadow the wave of reconciliation as the biggest issue now is the escalating tensions between Iran and Israel.
Wang Jin, an associate professor at the Institute of Middle Eastern Studies at Northwest University in Xi'an, believes the attack on Haniyeh could end political negotiations between Israel and Hamas.
"The ongoing dialogues are at a very critical stage, and this incident could negatively impact the direction of the Israeli-Palestinian situation," Wang said.
The death of Haniyeh is considered the "first most significant Israeli operation" since October 7, 2023, according to CNN, as the senior Hamas official was considered as a key interlocutor in hostage negotiations and a cease-fire deal in Gaza. His assassination will have a significant influence on negotiations.
The assassination of Haniyeh occurred one day after Israel claimed that a strike in Beirut, Lebanon on Tuesday had killed Hezbollah's most senior military commander, CNN said.
Israel is engaged in multi-front operations, striking Hezbollah in Lebanon on one side and launching attacks on Houthi forces in Yemen on the other. This latest strike within Iran's borders further highlights the escalating wave of conflicts in the Middle East, Sun Degang, director of the Center for Middle Eastern Studies at Fudan University, told the Global Times on Wednesday.
"As a result, reconciliation efforts between Israel and Palestine as well as peace talks between Hamas and Israel are likely to face significant setbacks," Sun said.
Further complications in relations
Some experts believe that Israel is currently adopting a two-pronged strategy.
"On one hand, it continues to carry out intermittent military strikes on Gaza. On the other, it is targeting Hamas' senior leaders through a policy of pinpoint elimination," Liu Zhongmin, a professor at the Middle East Studies Institute of Shanghai International Studies University, told the Global Times on Wednesday.
Since October last year, a number of senior Hamas officials have been killed as Israeli Prime Minister Benjamin Netanyahu vowed to eliminate Hamas, according to media reports. Other members of Haniyeh's family were killed by an Israeli air strike in June.
"This could lead to further complications in the relationship between Israel and Iran," Liu told the Global Times. Since the start of the latest Israeli-Palestinian conflict, tensions have also escalated between Hezbollah and Israel. Whether it is Hamas, Hezbollah or the Houthi rebels, they all receive a certain level of support from Iran, Liu noted.
"Although the military situation in southern Gaza has somewhat eased, the conflict between Hezbollah in Lebanon and Israel is escalating," Liu said. "The assassination of Haniyeh in Tehran has increased the risk of further conflict between Israel and Iran."
China's Special Envoy on Middle East Affairs Zhai Jun met with Iranian Ambassador to China Mohsen Bakhtiar on Tuesday.
The two sides agreed on the need to de-escalate tensions in southern Lebanon as quickly as possible to prevent the conflict from expanding or evolving into a regional war, according to a readout released by China's Ministry of Foreign Affairs on Wednesday.
Critical moment
With the death of Haniyeh, Hamas may face a leadership crisis, and there is bound to be significant internal struggles before new leaders emerge, some experts said, noting that the situation could put the entire organization at risk of division, further weakening its ability to act collectively.
"Hamas' power within the Palestinian factions has been weakened, raising new questions about who will govern the Gaza Strip in the future," Wen Shaobiao, an expert from the Shanghai International Studies University, told the Global Times on Wednesday.
"Based on the current situation in Gaza, the main armed forces of Hamas have been largely dismantled by the Israel Defense Forces, making it unlikely that Hamas can maintain control over the Gaza Strip. It is possible that new factions may emerge to fill the void," Wen told the Global Times.
The fate of Hamas has reached a very severe stage, as the organization's elite forces have suffered significant losses, Liu said.
"With the elimination of its top leader, Hamas faces the challenge of how to rebuild its organizational system," Liu said, noting that given the extent of damage to both its military and political structures and its leadership, Hamas is in a dire state.